Scaling up bioreactors is often one of the most complex steps of a biologic production process. We discuss common challenges—and how manufacturers can use CFD software to solve them, getting compounds to market faster.
Many biopharmaceutical manufacturers develop and optimize biologic processes in benchtop bioreactors with operating volumes smaller than 5 L. However, most production-scale bioreactors have volumes measured in thousands to tens-of-thousands of liters. So, when scaling up bioreactors from lab-scale to production-sized vessels, challenges arise. Why?
Challenges of Scaling Up Bioreactors
In this blog, we are following an example of scaling up bioreactors specifically within biologic production processes, which poses a unique set of challenges.
1. Living Cells
During the biologic production process, manufacturers produce drugs with living cells growing within stirred tank bioreactors. Because these are living organisms, they require a continuous and uniform supply of dissolved oxygen concentration, a fluid property that impacts cell growth, metabolism and protein quality. So, engineers had to design these bioreactors to realize specific chemical and fluid environments that maximize process yield.
The bioreactors are designed for specific scales and conditions, which means manufacturers need to understand how the process they developed and optimized at a small scale will translate to the production scale while still maintaining optimal bioreactor operation. The problem is that the complex fluid mechanics of this process make predicting how it will work at different scales difficult.
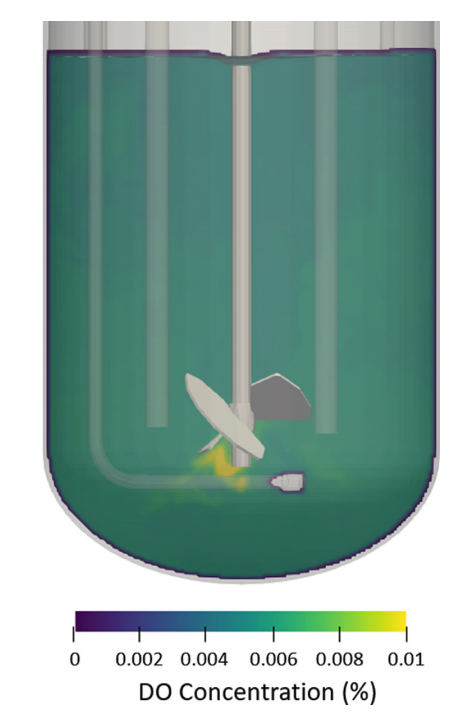
2. Complex Fluid Mechanics
Dissolved gas concentrations—a key environmental parameter in a biologic process—are informed by a complex convolution of blend time, energy dissipation rate, gas injection rate and bubble size. It is generally not possible to uniformly match these physics across all scales.
Also, with cultures that have high cell densities, the dissolved gas concentrations are likely to vary across the fluid, complicating the process further.
3. Slow, Difficult & Expensive Mathematical Modeling
The challenges of scaling up bioreactors increase as manufacturers consider different approaches to predict the fluid mechanics.
Most commonly, manufacturers use numerical modeling to understand transport processes in single-phase biopharmaceutical manufacturing devices. However, given the complex fluid mechanics within a biologic process with living organisms, a single-phase fluid model is not applicable.
To properly capture the complexity of cell culture processes, manufacturers must develop a two-phase fluid model that is capable of not only handling agitation and gassing but also supporting species transport across the bubble and liquid interface.
While a time-averaged approach—solving fluid motion with Reynolds-Averaged Navier Stokes (RANS) equations—is possible, the runtimes of these simulations are not practical within industrial research and design timescales.
Scaling Up Bioreactors with CFD Software:
Three Steps
So, to predict complex, multi-fluid mixing processes, biopharmaceutical manufacturers need a different type of approach: one that pairs lattice-Boltzmann-based transport algorithms with GPU resources.
This method—achieved with computational fluid dynamics (CFD) software—allows manufacturers to build time-dependent, bubble-resolved, two-phase models for investigating the real-time blending and oxygen transfer in bioreactors. And it generates predictions faster and with fewer modeling assumptions than a RANS approach in a multi-CPU environment. The results are in good agreement with experimental data with no model reparameterization between scales or operating conditions.
To learn how it works, follow these three steps.
1. Identify Relevant Conservation Laws
To begin, you first must identify relevant conservation laws that govern fluid, bubble and species transport within an agitated tank.
In the example we’re following, the manufacturer emphasized transport at the individual bubble level. They considered:
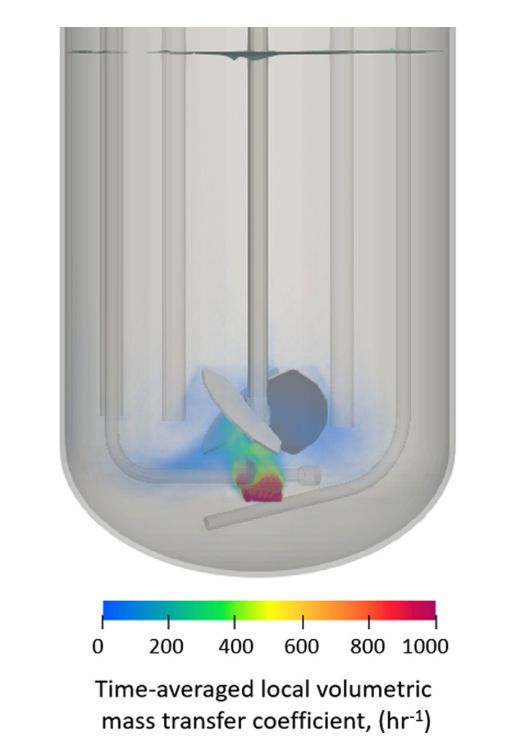
- Fluid mechanics
- Energy input and dissipation
- Bubble dynamics
- Mass transfer
- Species transport in the fluid
To model these, they identified two corresponding transport equations:
- Navier-Stokes: Models the conservation of momentum of a fluid particle
- Boltzmann transport: Models the conservation of transport carrier probability density
2. Solve Transport Equations
To solve the transport equations identified in step #1, you can use a combination of the lattice-Boltzmann method (for the fluid) and Newtons second law (for the bubble trajectories). But to build the model that solves these equations, you need CFD software that supports the lattice-Boltzmann method.
Modern CFD software solves the lattice-Boltzmann algorithms on GPUs, which, compared to traditional finite element and finite difference approaches, provides massive improvement in computational speed when solving these fluid transport equations.
The trend is amplified in the GPU-based environment because GPUs can fully exploit the localize nature of the Boltzmann transport equation. As a result, the runtime performance realized on a desktop GPU is faster than what can be realized on a CPU cluster—and thus more industrial practical.
Interested in reading more details about using CFD to predict mass transfer in bioreactors? Check out our academic paper with Bristol Myers Squibb.
READ MORE
In this scenario, the manufacturer used CFD software to model several systems at multiple scales, including:
- Blend time at 500 L and 2000 L
- Power input and dissipation at 500 L and 2000 L
- Velocity field and local energy dissipation rates at 5 L
- Mass transfer coefficient at 5 L, 200 L, 500 L and 2000 L
- Sensitivity of output to coalescence and break-up models at 5 L
3. Validate CFD Model
Before you can apply the models discussed above, you have to validate it by comparing the predicted values to experiments and measured data across multiple reactor sizes and operating conditions.
Once validated, the approach can be repeated and even extended to similar applications.
In this scenario, the approach can be extended to handle multispecies transport and include combined oxygen transport with carbon dioxide scrubbing. Further, you can incorporate reactions into the conservation of species equation and solve them in real-time. Used in this way, you can generalize the approach to model nutrient consumption and drug product growth.
Ultimately, because the fluid, species and bubble transport physics are solved in tandem and in real-time, this modeling approach can be used to study time evolving, multi-physics production rates.
Conclusion
Luckily, the problem addressed above has already been modeled, solved and validated with M-Star CFD and Bristol Myers Squibb.
This means you can use this mechanistic approach for predicting mass and oxygen transfer—and scaling up bioreactors—for your own needs right away. This approach has proven to help pharmaceutical companies speed up the timeline and reduce the cost of biologics production, so they can get compounds to market faster.
If scaling up bioreactors is holding up your biologic production process, M-Star can help. Contact us for a demo or free trial.